Energy IQ: What is a solid oxide fuel cell and how fuel cells work
By Aytek Yuksel, Content Marketing Leader - Power Systems
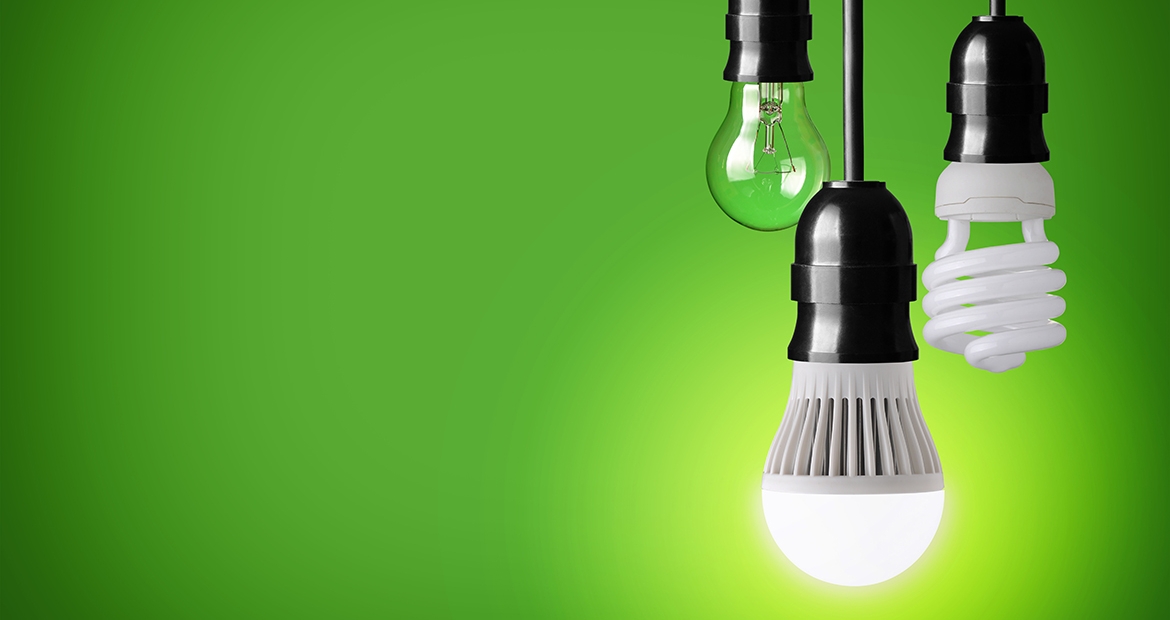
July 20, 1969 might not immediately ring a bell, but what if we were to give you a hint by saying the word "Apollo?"
Yes, that was the date humans first landed on the moon as a part of the Apollo 11 mission. Most of us remember details such as the images of astronauts on the Moon’s surface, and Neil Armstrong’s reaction, “That's one small step for [a] man, one giant leap for mankind.” Yet many don’t know how the spacecraft obtained its electrical power through this historical mission.
Fuel cells were NASA’s answer to this challenge, as Apollo spacecraft carried three hydrogen-fuel cells to provide electricity for all the equipment. Each of these fuel cell modules had 31 individual fuel cells stacked together 1.
Over 50 years later, fuel cells today are used in a variety of applications ranging from vehicles to data centers. Let’s focus on solid oxide fuel cells and answer four common questions to boost your energy IQ.
Question No. 1: What is a solid oxide fuel cell?
Simply put, all fuel cells are energy converters; they convert energy from one form to another. More specifically, fuel cells convert the chemical energy stored in the fuel to electric and thermal energy (heat), without the need for combustion. Engines and power plants also convert energy from one form to another, but they rely on combustion, which reduces the overall efficiency of the energy conversion.
Solid oxide fuel cells are one of the many types of fuel cells and produce electricity, water, heat and small amounts of carbon dioxide using natural gas as the fuel.
Question No. 2: How does a solid oxide fuel cell work?
Electricity is the movement of electrons, and all elements (hydrogen, oxygen and others) have varying numbers of electrons.
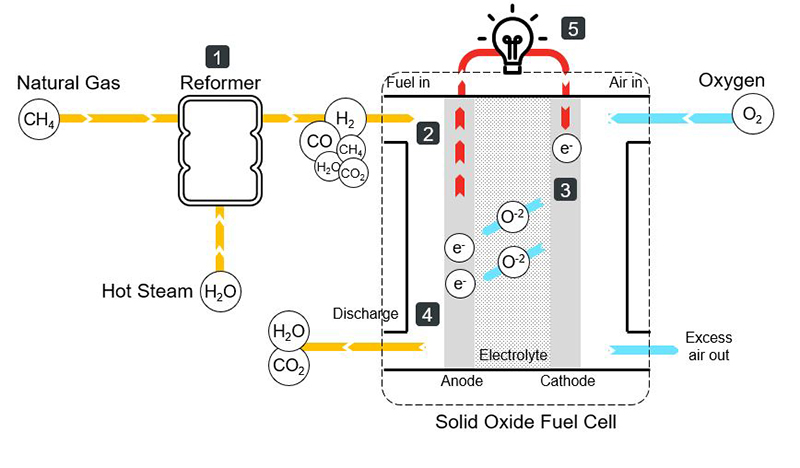
A solid oxide fuel cell utilizes the movement of electrons and generates electricity in few basic steps.
- Natural gas goes through a steam-reforming process. This chemical reaction produces hydrogen (H2), carbon monoxide (CO), carbon dioxide (CO2) and steam (H2O). There will be some unreformed natural gas left in the mix as well.
- The mix of elements from the reformer enter the fuel cell at the anode side. Meanwhile, air (including oxygen) enters the fuel cell at the cathode side.
- Oxygen in the air combines with free electrons to form oxide ions at the cathode. Oxide ions with free electrons travel from the cathode to the anode through the electrolyte.
- At the anode, oxide ions react with hydrogen forming water (steam) and with carbon monoxide (CO) forming carbon dioxide (CO2).
- Reactions covered on Step #4 release free electrons. These free electrons travel to cathode through the external electrical circuit, producing electricity.
Question No. 3: What are the differences between solid oxide and proton exchange membrane fuel cells?
Proton exchange membrane (PEM) fuel cells, also known as hydrogen fuel cells, and solid oxide fuel cells share the same basic operating principles yet have many differences. Here are two of these differences impacting how these technologies are being used today.
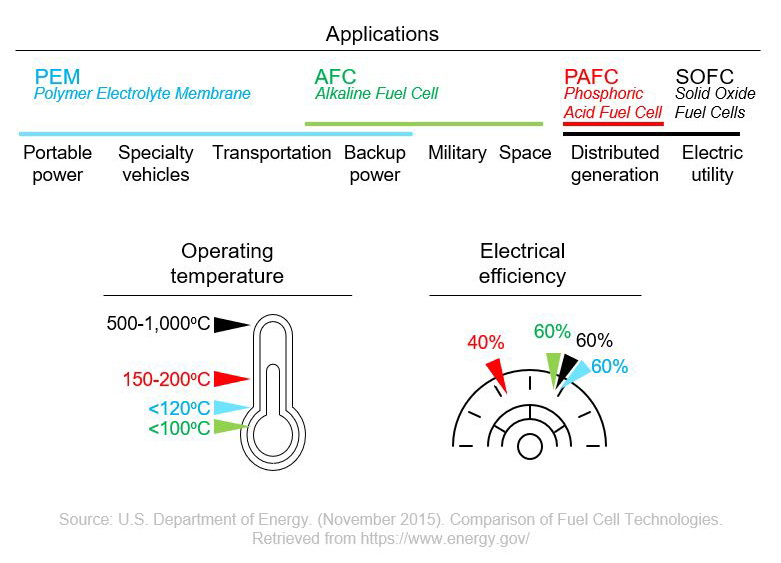
- Fuel : PEM fuel cells use pure hydrogen (H2) as fuel. Meanwhile, solid oxide fuel cells can use hydrocarbon fuels such as natural gas, methane and propane to produce electricity.
- Size: While a single cell of a PEM fuel cell and a solid oxide fuel cell don’t differ significantly in size, the size difference comes into play when a fuel cell module is assembled together. A typical PEM fuel cell module would be smaller than a solid oxide fuel cell module. This makes PEM fuel cells a good candidate for transportation applications ranging from trucks and buses to trains and boats.
Question No. 4: Why do we need solid oxide fuel cells?
The benefits of solid oxide fuel cells vary depending upon the application but two benefits remain consistent across applications.
- High efficiency delivers environmental and financial benefits: Electrical efficiency of solid oxide fuel cells reach up to 60% 2. This means 60% of the energy stored in the fuel is converted to useful electrical energy. This is much higher than the efficiencies of coal power plants. Moreover, the use of excess heat produced by the fuel cell for heating purposes in a cogeneration application will further increase the overall efficiency over 80%. Additionally, since fuel cells could be located locally, they eliminate the inefficiencies associated with distribution losses from large central power plants.
This high efficiency delivers financial benefits and minimizes the environmental footprint, since solid oxide fuel cells commonly use natural gas as fuel in comparison to traditional power plants using coal as fuel. Solid oxide fuel cells also don’t emit sulphur oxides and particulate matter.
- Modular design brings scalability: The individual fuel cells are bundled together to form a stack. These stacks are then combined with other equipment to form modules. These individual power generation modules can be paralleled to form the fuel cell power system. You can add more fuel cell modules to the overall system as you need. This provides financial flexibility for the user to align the power generation investments with business needs.
Microgrids and fuel cells to energy storage devices, our energy future includes a diverse set of technologies and fuels, and Cummins is committed to innovating and delivering a variety of power solutions to meet these diverse needs of customers.
Sign up below for Energy IQ to periodically receive relevant insights and trends about energy markets. To learn more about distributed generation solutions Cummins offers, visit our webpage.
Think your friends and colleagues would like this content? Share on LinkedIn and Facebook.
References:
1 Smithsonian National Air and Space Museum. (n.d.). Apollo to the Moon, About the Spacecraft [Web page]. Retrieved from https://airandspace.si.edu/
2 U.S. Energy Department, Office of Energy Efficiency and Renewable Energy . (n.d.). Comparison of Fuel Cell Technologies [Table]. Retrieved from https://www.energy.gov/
Author Profiles
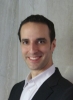
Aytek Yuksel, Content Marketing Leader - Power Systems
Aytek is a marketing leader at Cummins, focusing on technology and thought leadership. Since joining in 2008, he has held various marketing roles and now shares insights on markets, technologies, and energy transition. Aytek lives in Minneapolis with his wife and two kids.
Related Topics
Related Tags